Research
Microbiomes are complex across scales – from stochasticity between single cells to disorder among hundreds of interacting species. This complexity is challenging to understand using traditional low-throughput correlational studies. Our lab overcomes this challenge by integrating theory and high-throughput experiments. Key theoretical approaches include mathematical models of ecological dynamics and their analysis using tools from statistical physics. Experimental approaches include in vitro assembly of gut microbiotas and their analysis using metagenomics and metabolomics. This combination will make microbiome engineering quantitative. Ongoing research projects include:
Dissecting microbial interactions
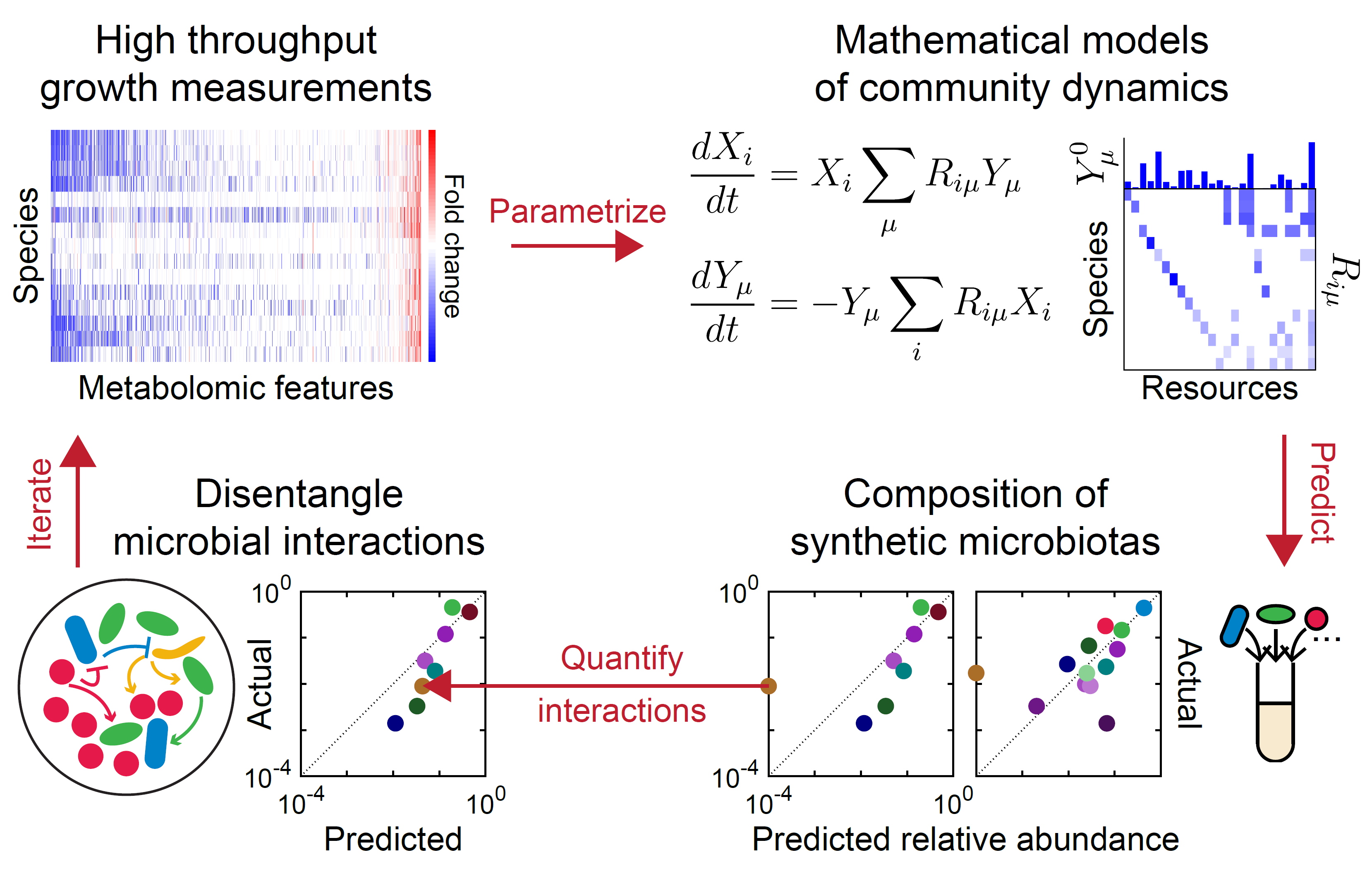
Microbes interact via a plethora of mechanisms, including resource competition, metabolic cross-feeding, and environmental modulation. We disentangle this complexity using community assembly experiments, metabolomics, metagenomics, and mathematical models.
- Metabolomic profiles of individual gut bacterial species allowed us to predict community dynamics in vitro (Ho et al, Nat Microbiol 2024). We are applying this approach to investigate microbial interactions in complex environments and during evolution.
- Antibiotic activity can affect the resource competition landscape and vice versa, and this interplay can result in synergism or antagonism among multiple antibiotics (Newton et al. Nat Commun 2023). We are testing these predictions and leveraging them to engineer community dynamics.
Engineering the human gut microbiome
To quantitatively engineer microbiome composition or function, we build theoretical and experimental tools with focus on the human gut microbiota.
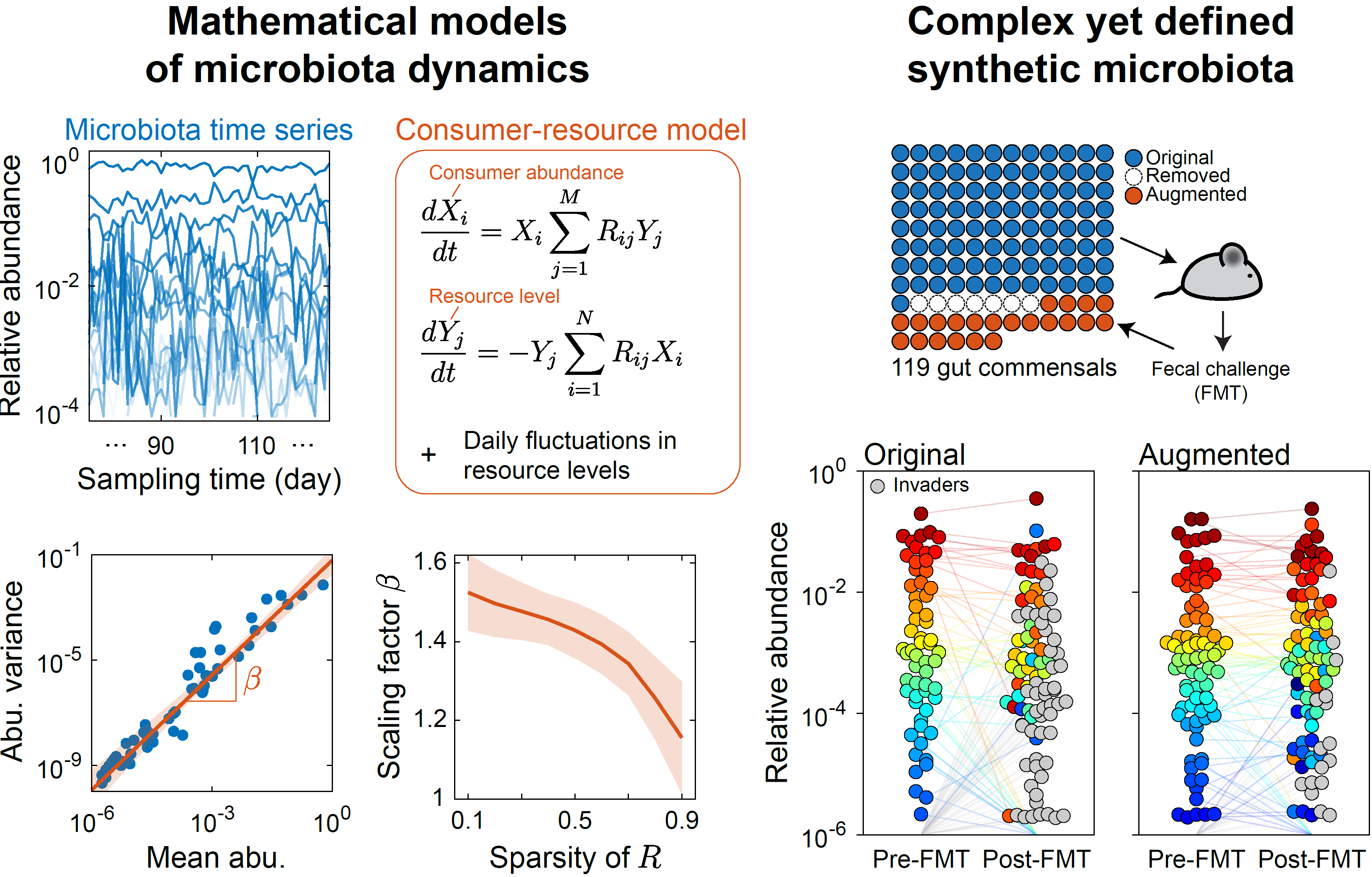
- Microbiomes exhibit special statistics of fluctuations in species abundances, and these fluctuations can be reproduced by a mathematical model of resource competition (Ho et al, eLife 2022). We are using this link to infer the ecological parameters of various ecosystems.
- A highly diverse yet defined model community reproducibly colonizes mice gut, and mice colonized by either the model community or human fecal communities are phenotypically similar (Cheng et al, Cell 2022). We are quantifying microbial interactions in this model system.
Microbial cell cycle regulation
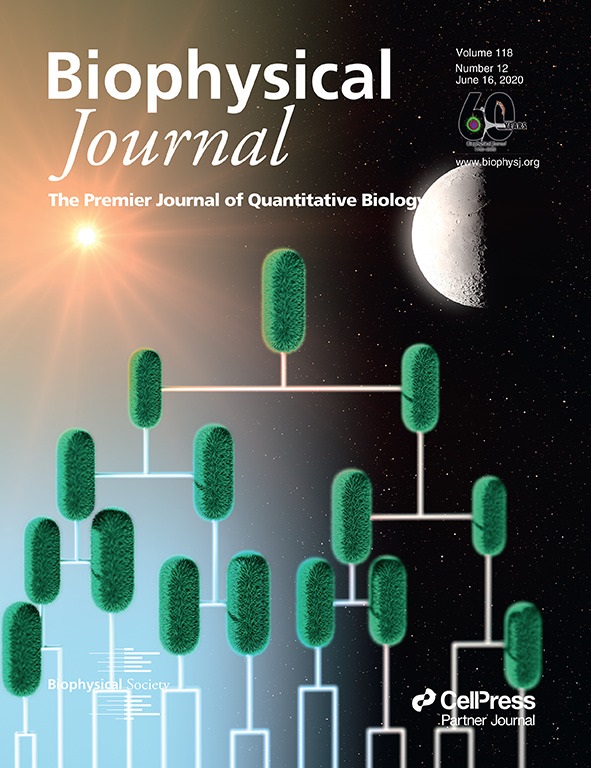
How cells regulate and coordinate their growth and cell cycle to maintain a homeostatic cell size is a fundamental question. We use microfluidic-enabled imaging to capture how single cells grow and divide in face of stochasticity, and analyze the data by constructing mathematical models of gene regulatory networks to understand the design principles underlying microbial cell cycle regulation.
- A simple quantitative law describes cell size control in microbes from all domains of life (Ho et al, Annu Rev Biophys 2018). How and why this striking generality arises remain outstanding open questions.
- Cyanobacteria also follows the general law identified above, except for a modification by the circadian clock (Ho et al, Biophys J 2020). How does this coupling affect population fitness?